Looking at life in some of the most extreme conditions on Earth, Oceans Across Space and Time graduate student Luke Fisher of Scripps Institution of Oceanography explores how microorganisms function in exotic environments on our own planet. This kind of research is key to helping scientists develop methods to search for life on other worlds.
In the February 2021 paper in Environmental Microbiology, “Current state of athalassohaline deep-sea hypersaline anoxic basin research-recommendations for future work and relevance to astrobiology”, lead author Fisher and a team of OAST investigators explore polyextremophiles – which are microbial communities that are adapted to multiple extremes (pressure, anoxia, salt stress, desiccation) – and how scientists can better understand the limits of life as we know it.
Polyextremophiles are found in places like the Kryos, Discovery, and Hephaestus basins in the Eastern Mediterranean Sea on Earth – these areas are known as deep-sea hypersaline anoxic basins (DHABs). Like the famous Dead Sea and the Great Salt Lake, salt deposits became concentrated in these areas when now-extinct oceans or seas evaporated. Later, exposure of these ancient evaporites, salts and minerals, dissolve; creating an extremely dense brine that collects in the deep-sea basins. Due to the lack of mixing, the brine quickly becomes anoxic.. DHABs are unique because they present a combination of stressors for life that aren’t found elsewhere: osmotic stress, anoxia, elevated hydrostatic pressure and low water activity.
“Too much salt causes cells to dehydrate and die, as osmotic pressure moves water from the cell to the salty environment ,” Fisher explained. This means that in dense brines like DHABs, very little water is available for life to use. “A lack of available water affects all the machinery of the cell, proteins, DNA replication, etc. All life needs water,” said Fisher.
The concentration of salts increases as depth increases in DHABs, and so do other stressors on microbial life, such as higher pressure and less available oxygen. It’s this combination of stressors that make DHABs one of the most extreme places we can find life on Earth.
At a certain point, the stressors become too much for microbial life, and not even polyextremophiles can survive. This cutoff point has been studied in places like the Kryos, Discovery, and Hephaestus basins, but Fisher points out in the paper that some inconsistencies in measurements need to be corrected in future work, in order to clarify the limits for life in DHABs.
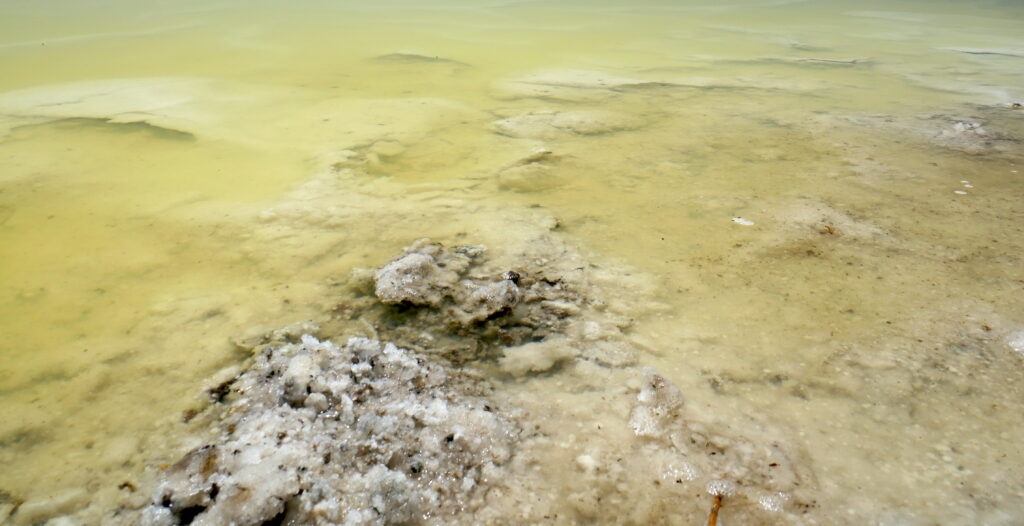
On the other side of the world, South Bay Salt Works in southern California is a company that harvests salt by allowing the sun to evaporate water from a series of ponds, leaving concentrated salt brine behind. By investigating salt-loving microbes from these ponds, especially ponds dominated by MgCl2, Fisher’s work with the OAST team is improving our understanding of how microbes can adapt and survive in extreme environments like DHABs.
“I find it interesting to work in these places, especially the MgCl2 brines, because they are much more rare,” Fisher said. “There might be life living here – and if they are, they likely have some crazy adaptations thereby changing our perception of life and what is considered biologically habitable.”
One way Fisher is using the SBSW pond samples to study extreme life on Earth, is by culturing salt-loving microbes in the absence of oxygen – one of the many stressors present in DHABs.
“Anaerobic microbes, meaning microbes that don’t use oxygen, are generally found in deeper sediment mud (usually black mud, black coloration generally means anoxia as chemical reactions in the absence of oxygen turn sediments black). I collected this type of mud at SBSW to try to culture methanogens, which are microbes that produce methane. We are interested in culturing halophilic methanogens – salt loving methane producing microbes – because they are relevant in DHAB environments. Being able to collect and grow them in-lab means we can learn more about how they survive and adapt,” Fisher said.
Not only does Fisher’s research improve our understanding of polyextremophiles on Earth, it could also further our understanding on where life might exist elsewhere in the universe.
“These sites are interesting because they offer windows to extraterrestrial environments (Martian brines for example) that teach us more about what these extraterrestrial environments might be like,” Fisher said.
Several moons that orbit Saturn and Jupiter are thought to harbor oceans beneath their icy surfaces. The presence of highly concentrated salts like magnesium and sulfur-rich compounds on Europa (one of Jupiter’s moons) means that understanding these exotic brines – like those dominated by MgCl2 at SBSW and in deep ocean basins – may be a crucial step in the search for life on other oceans across space and time. Moreover, evaporite basins exist on present day Mars, such that work in DHABs and SBSW can help reveal what happened to these environments—and any microbes that may have once lived there–as Mars lost its water.